All human cells are enclosed in a greasy membrane that is embedded with thousands of different proteins. These so-called membrane proteins carry out countless functions, from regulating our blood pressure, to coordinating our immune responses, to controlling the firing of neurons in our brains. Membrane proteins are so important that they are targeted and regulated by more than half of all pharmaceutical drugs on the market today.
Membrane proteins are first manufactured inside the cell and transported to an organelle called the endoplasmic reticulum (ER). There, the proteins are inserted into the membrane, where they carry out myriad functions. For many membrane proteins, this insertion process relies on a complicated structure known as the ER membrane protein complex (EMC). The EMC is evolutionarily ancient, found broadly in eukaryotic cells from fungus to humans. And it is exploited by viruses (potentially including the novel coronavirus responsible for the COVID-19 pandemic) which use it to construct and transport some of their own viral proteins in an infected organism. Thus, understanding how the EMC works is an important part of understanding basic cellular biology and could offer clues about how to inhibit diseases.
Now, Caltech scientists have seen the EMC for the first time, with a technique called single-particle cryo-electron microscopy—through which samples are frozen, imaged at the atomic level, and then computationally reconstructed in fine detail.
The research was done in the laboratory of Rebecca Voorhees, assistant professor of biology and biological engineering and Heritage Medical Research Institute Investigator. A paper describing the study appears in the journal Science on May 21.
The EMC was discovered about 10 years ago, but until recently, no techniques were available that could image its structure. When Voorhees arrived at Caltech in 2017, one of her first goals was to work in collaboration with Caltech's cryo-electron microscopy facility (one of the few such facilities in the world) to understand how membrane proteins are made.
"Solving the structure enables answering questions like, at the molecular level, how does the EMC insert proteins into cell membranes? How can it interact with so many different proteins? What happens when these processes go wrong in the case of disease?" Voorhees explains.
The new structural model of the EMC shows how its nine different essential subunits come together, although the functions of some of the subunits are still not understood. The model suggests that the EMC may do more than just help to guide and insert proteins into the cell membrane; it may actually help proteins to fold and assemble properly and may conduct "quality control" checks. Voorhees and her team now aim to design experiments to investigate the functions of the complex's different components.
"In structural biology, we often see ourselves as explorers with biological targets being mountaintops to be reached," says Professor of Biochemistry Bil Clemons, a structural biologist at Caltech who was not involved in the study. "Since its discovery, the EMC has been a mountain of obvious importance, and it is thrilling to finally see it in all of its glory. It is a testament to the talent of Professor Voorhees and the success of Caltech at providing the resources that make it possible. This structure is a landmark in the field of membrane protein biology."
"Structural biologists often like to reference a particular Richard Feynman quote—to paraphrase, 'If you want to understand fundamental biological questions, just look at the thing.' Caltech's cryo-EM facility, and the teamwork of my truly tremendous lab members, really made this work possible," says Voorhees.
The paper is titled "Structural basis for membrane insertion by the human ER membrane protein complex." Caltech postdoctoral scholar Tino Pleiner, graduate student Giovani Pinton Tomaleri, and staff scientist Kurt Januszyk are the study's co-first authors. In addition to Voorhees, other co-authors are postdoctoral scholar Alison Inglis and graduate student Masami Hazu. The research utilized the Caltech High Performance Computing Center and the Caltech Cryo-EM Facility. The work was funded by the Heritage Medical Research Institute, the Kinship Foundation, the Pew-Stewart Foundation, and the National Institute of General Medical Sciences of the National Institutes of Health.
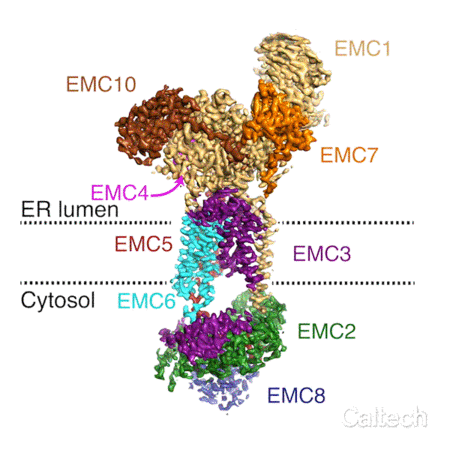
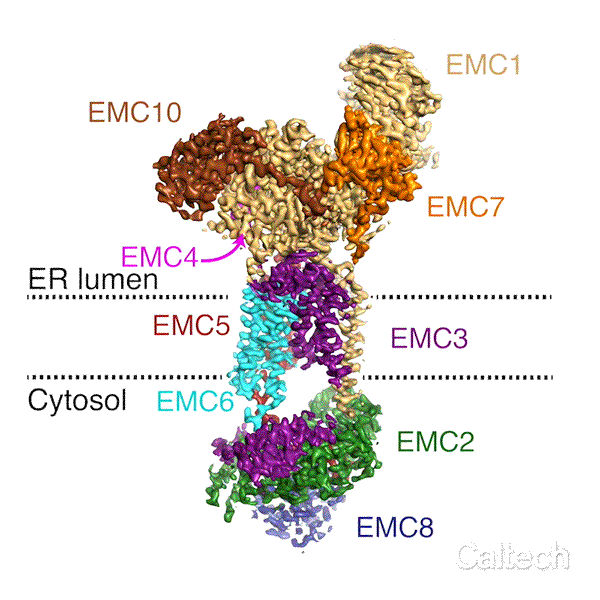