A new study provides a comprehensive look at the motions and frictional laws driving destructive thrust-fault earthquakes
Simulating an earthquake on a miniature scale in a laboratory known unofficially as the "seismological wind tunnel," engineers and seismologists have produced the most comprehensive look to date at the complex physics of friction driving destructive thrust-fault earthquakes.
Thrust-fault earthquakes occur when one side of a fault slides over or under the other side. Thrust faults have been the site of some of the world's largest quakes, including the 2011 Tohoku earthquake off the coast of Japan, which generated a tsunami that damaged the Fukushima nuclear power plant.
However, the motion or forces that cause these earthquakes cannot be directly measured at the source, since much of the action takes place deep inside the earth. To gain more insight into them, a team of researchers created and observed thrust-fault earthquakes at a unique "laboratory earthquake" facility at Caltech.
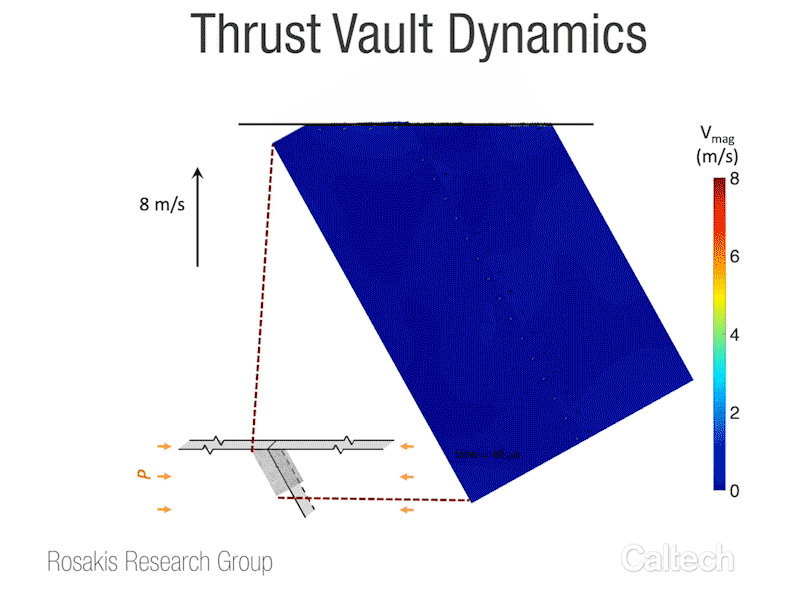
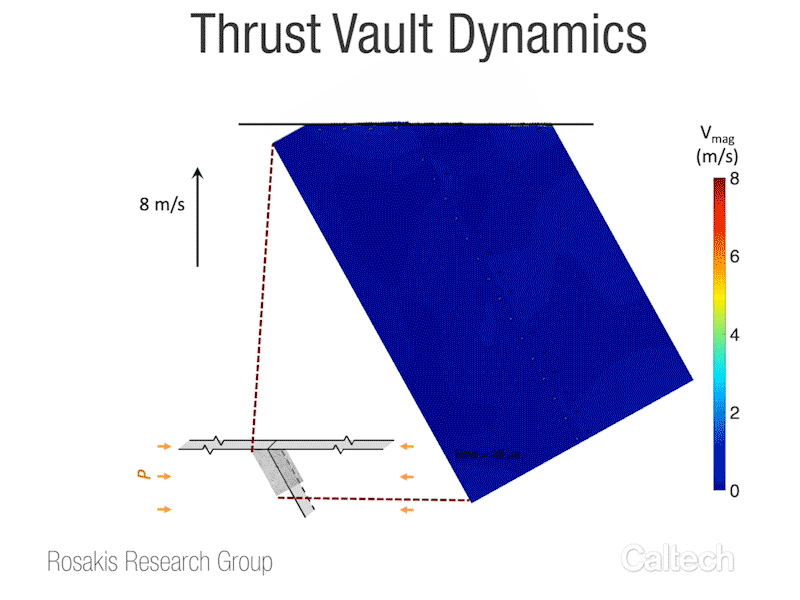
"Simulating earthquakes in a lab lets us observe how these brief and violent events grow and evolve by ‘slowing down' their motion through high-speed photography and optics," says Ares Rosakis, the Theodore von Karman Professor of Aeronautics and Mechanical Engineering, who runs the facility and has introduced the concept of laboratory earthquakes together with former Caltech Seismology Laboratory director Hiroo Kanamori, John E. and Hazel S. Smits Professor of Geophysics, Emeritus.
Rosakis is the corresponding author of a paper on the new research that was published by the Proceedings of the National Academy of Sciences on August 25. He co-authored this paper with Nadia Lapusta, the Lawrence A. Hanson, Jr., Professor of Mechanical Engineering and Geophysics, his longtime collaborator on problems at the interface between engineering and earthquake science; former Caltech postdoctoral scholar Yuval Tal, currently an assistant professor at Ben-Gurion University of the Negev in Israel; and Caltech research scientist Vito Rubino.
To create an earthquake in the lab, the team first cut in half a transparent block of a type of plastic known as Homalite, which has similar frictional properties to rock. They then put the two pieces together under pressure and shear, simulating tectonic pressure that slowly builds up along a fault line. Next, they placed a small wire fuse at the location under a simulated Earth surface where they wanted the quake to originate. Triggering the fuse reduced friction at that location and allowed a very fast rupture to initiate and propagate up the miniature fault toward the free surface of the Earth, producing intense shaking. Meanwhile, high-speed imaging technology recorded the evolution of stresses, and thus of the friction coefficient, along the fault as the rupture approached the free surface—one millionth of a second at a time.
The "seismological wind tunnel" has been around since 1999, but the addition of digital image correlation (DIC) in 2015 has given the engineers "a new pair of eyes," Rosakis says. DIC measures minute shifts in the location of individual points throughout a material over time, indicating how strain and stress dynamically evolve throughout the material during a simulated earthquake. With that information, Rosakis and his colleagues were able to map how a rupture moves up a fault, interacts dynamically with the ground surface, and even affects itself through dynamically propagating waves generated by every motion.
They noted a very rapid change in "fault-normal" stress, which is the compressive force that keeps the fault clamped shut. There are a number of reasons why the fault-normal stress may vary when the fault slips. In the case of thrust-fault earthquakes, the researchers noted that the fault-normal stress went through a rapid cycle of increasing and decreasing amplitude because waves emitted by the rupture were then reflected off of the simulated Earth surface like an echo.
Because this stress, which normally keeps a fault locked in place, was rapidly changing in strength, it altered the fault's resistance to slipping, known as shear motion. When the fault-normal stress ebbs, the fault is clamped less tightly in place and becomes more likely to slip, causing a quake.
Most importantly, the researchers were able to challenge a commonly accepted (but also disputed) assumption that friction locking the plates in place along a fault is always proportional to the fault-normal stress. What they found instead is that, as the rupture interacts with the earth's surface, there is a significant time lag between changes in fault-normal stress and the resulting shear resistance, and the two are not proportional on the time scale of the rupture process.
"This implies the presence of a complex history-dependent mechanism governing friction in the presence of rapid fault normal stress, which are characteristic of thrust-fault configurations," Rosakis says.
"While discrepancy between changes in normal stress and friction has been pointed out by prior studies, it has not been clear how significant this effect is for thrust earthquakes," adds Lapusta. "Our measurements showed that the effect is much larger than could be expected based on prior studies and allowed us to improve the existing friction laws."
The team hopes that these physical insights into the dynamics of an earthquake can help geoscientists create more accurate computer models of earthquake ruptures propagating along real-world thrust faults.
"Getting the frictional resistance and, hence, the simulated motion right next to the earth's surface is especially important, since it significantly influences ground shaking as well as tsunami generation if the fault trace happens to be under water," Lapusta says. "Indeed, many destructive earthquakes occur as thrust ruptures in subduction zones, sometimes causing devastating tsunamis such as during the 2011 magnitude 9.0 Tohoku earthquake."
"The history-dependent frictional law of the fault, which is very hard to determine, is any modeler's biggest assumption," Rosakis says. "Now we have one more piece of the puzzle pinned down."
The paper is titled "Illuminating the physics of dynamic friction through laboratory earthquakes on thrust faults." This research was supported by the National Science Foundation, the U.S. Geological Survey, the Caltech Mechanical and Civil Engineering (MCE) department's Big Ideas Fund, Caltech's Division of Geological and Planetary Sciences, and the Southern California Earthquake Center.