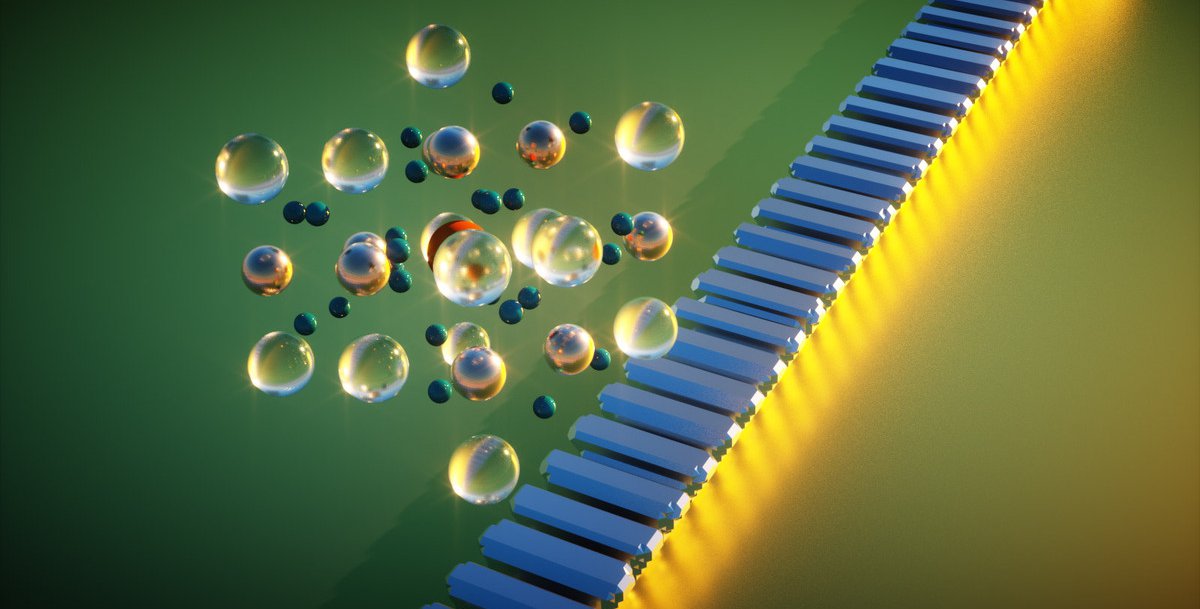
Still "Plenty of Room at the Bottom": Nanoscience at Caltech Twenty Years On
April 03, 2024
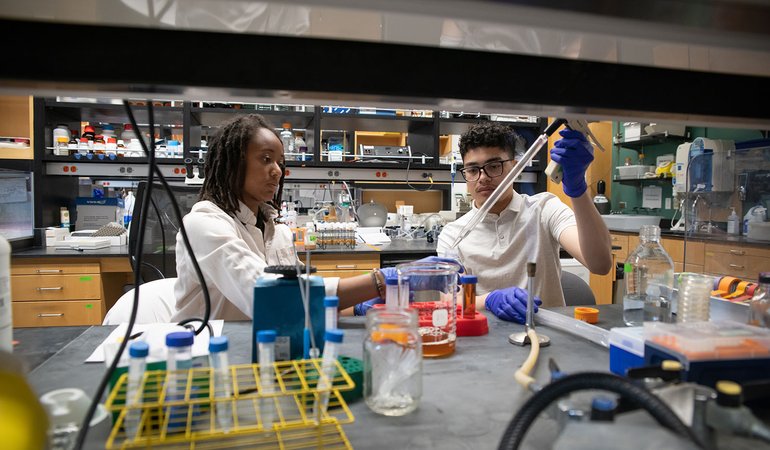
Nanophotonics
In 2009, KNI resarchers created a optomechanical crystal capable of trapping light and sound vibrations together, opening up the opportunity to send large amounts of information and to detect and weigh individual macromolecules. The creation of tiny optical cavities has allowed scientists to produce light waves, known as solitons, that circulate indefinitely rather than dissipating. These tiny boxes of light have been engineered at the KNI with periodic nano-patterning that causes rare-earth ytterbium ions to bounce back and forth such that photon emissions can be detected and collected. In the process of working with ytterbium, a new phenomenon was discovered: atoms that are transparent to certain frequencies of light. A laser's light will usually bounce off an atom, but as the frequency is adjusted, it can create what researchers call "collectively induced transparency." Just how this new area of physics operates is still under study.
In 2009, KNI resarchers created a optomechanical crystal capable of trapping light and sound vibrations together, opening up the opportunity to send large amounts of information and to detect and weigh individual macromolecules. The creation of tiny optical cavities has allowed scientists to produce light waves, known as solitons, that circulate indefinitely rather than dissipating. These tiny boxes of light have been engineered at the KNI with periodic nano-patterning that causes rare-earth ytterbium ions to bounce back and forth such that photon emissions can be detected and collected. In the process of working with ytterbium, a new phenomenon was discovered: atoms that are transparent to certain frequencies of light. A laser's light will usually bounce off an atom, but as the frequency is adjusted, it can create what researchers call "collectively induced transparency." Just how this new area of physics operates is still under study.
Credit: Lance Hayashida/Caltech
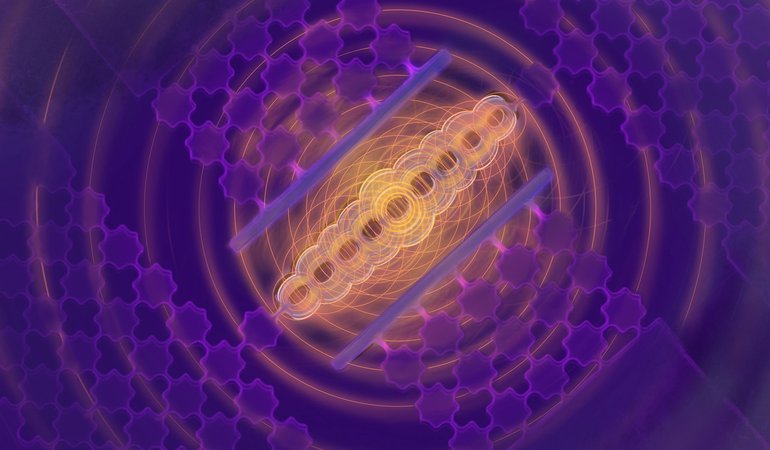
Quantum Information
Within the limits of ordinary human senses, the material world can be explained quite well by classical physics. But once it became possible to detect phenomena at the atomic and subatomic level, quantum mechanics was required to describe these phenomena. As nanoscale science progresses, it has become possible to not only observe atomic and subatomic behaviors, but to manipulate them with nanoscale devices. This has opened the door to the possibility of quantum computing, which is expected to solve problems that are impossible for conventional computers. KNI researchers are experimenting with a variety of ways to store quantum information, including through sound waves (phonons), light waves (photons), nuclear spin waves, and surface plasmons (the sea of electrons bridging the space between a metal surface and the material above it).
Within the limits of ordinary human senses, the material world can be explained quite well by classical physics. But once it became possible to detect phenomena at the atomic and subatomic level, quantum mechanics was required to describe these phenomena. As nanoscale science progresses, it has become possible to not only observe atomic and subatomic behaviors, but to manipulate them with nanoscale devices. This has opened the door to the possibility of quantum computing, which is expected to solve problems that are impossible for conventional computers. KNI researchers are experimenting with a variety of ways to store quantum information, including through sound waves (phonons), light waves (photons), nuclear spin waves, and surface plasmons (the sea of electrons bridging the space between a metal surface and the material above it).
![SSPD-1440x1060pixels[48]](https://caltech-prod.s3.amazonaws.com/main/images/SSPD-1440x1060pixels48.2e16d0ba.fill-770x450-c100.jpg)
New Energy Solutions
It has become imperative to develop alternative energy sources, such as solar and wind power, that are efficient, safe, and renewable, and that minimize waste. To that end, Caltech researchers, using KNI's instruments, have made pioneering strides in something quite new: space-based solar power. The Space Solar Power Project, funded by the Donald Bren Foundation, at first anonymously in 2013, and then publicly in 2021, is working toward harnessing solar power with space-based solar collectors and beaming it back to Earth 24 hours a day, without interference from weather or darkness. A demonstration mission was launched in January 2023, and by May 2023, power was wirelessly transmitted from space for the first time to a receiver on the roof of the Gordon and Betty Moore Laboratory of Engineering on the Caltech campus. Nanomaterials are also being pioneered to extend the lifetime of rechargeable lithium batteries, and to make them more energy-dense.
It has become imperative to develop alternative energy sources, such as solar and wind power, that are efficient, safe, and renewable, and that minimize waste. To that end, Caltech researchers, using KNI's instruments, have made pioneering strides in something quite new: space-based solar power. The Space Solar Power Project, funded by the Donald Bren Foundation, at first anonymously in 2013, and then publicly in 2021, is working toward harnessing solar power with space-based solar collectors and beaming it back to Earth 24 hours a day, without interference from weather or darkness. A demonstration mission was launched in January 2023, and by May 2023, power was wirelessly transmitted from space for the first time to a receiver on the roof of the Gordon and Betty Moore Laboratory of Engineering on the Caltech campus. Nanomaterials are also being pioneered to extend the lifetime of rechargeable lithium batteries, and to make them more energy-dense.
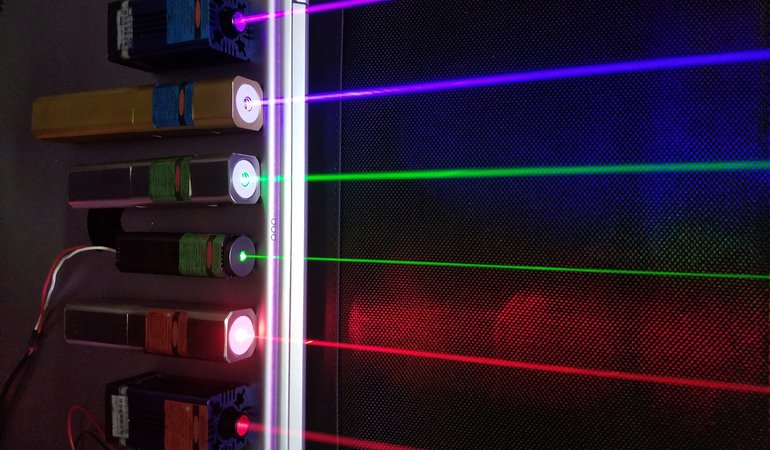
Electronics of the Future
The information age would not have been possible without microchips—semiconductor transistors on silicon chips that drive digital electronics. In 1965, Gordon Moore (PhD '54) predicted that the number of transistors that could fit on a microprocessor would double every 18 months to 2 years, and for decades, technology has met or exceeded that rate. Now the chase is on to identify new materials that can support faster, smaller, lighter, and more powerful electronic capabilities without thermal degradation from the power output. Several candidates are being investigated at the KNI, including graphene, a one-atom thick sheet of graphitic carbon that is an excellent electrical and thermal conductor; metasurface optics that manipulate light between tiny folded surfaces; electro-optical frequency division that uses a pair of laser beams to create precise frequencies that can be used as time standards; and optical frequency combs that can substitute multiple lasers, each operating at a specific frequency, for a single multifrequency optical source the size of a match box.
The information age would not have been possible without microchips—semiconductor transistors on silicon chips that drive digital electronics. In 1965, Gordon Moore (PhD '54) predicted that the number of transistors that could fit on a microprocessor would double every 18 months to 2 years, and for decades, technology has met or exceeded that rate. Now the chase is on to identify new materials that can support faster, smaller, lighter, and more powerful electronic capabilities without thermal degradation from the power output. Several candidates are being investigated at the KNI, including graphene, a one-atom thick sheet of graphitic carbon that is an excellent electrical and thermal conductor; metasurface optics that manipulate light between tiny folded surfaces; electro-optical frequency division that uses a pair of laser beams to create precise frequencies that can be used as time standards; and optical frequency combs that can substitute multiple lasers, each operating at a specific frequency, for a single multifrequency optical source the size of a match box.
Credit: 彭嘉傑 via Wikimedia Commons
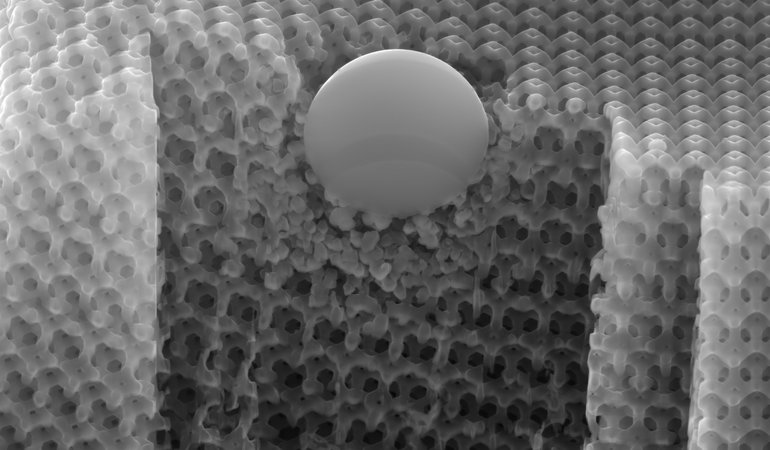
Nano-architected materials and devices
One reason why KNI's establishment and continued operation is so widely celebrated in science is that researchers have the ability to use the facility to create new materials, or old materials in new configurations with different capabilities. One such nano-architected material, built from tiny carbon struts, is more impact resistant than Kevlar. Other nano-architected materials have the capacity to refract light backward, a phenomenon not found in nature, and to create metamaterials that can be tuned to take on a variety of shapes. Known materials, such as a sponge, have the ability to take on different shapes depending on conditions: one shape when wet, another when dry. But these carefully architected lattices can be finely controlled to take on a variety of "in-between" states. Some nanoscale devices have benefited from machine learning, where multiple iterations of a design are printed out until the device is optimized to, for example, separate light by wavelength and polarization, a technology that could be added to cameras to increase their resolution and efficiency.
One reason why KNI's establishment and continued operation is so widely celebrated in science is that researchers have the ability to use the facility to create new materials, or old materials in new configurations with different capabilities. One such nano-architected material, built from tiny carbon struts, is more impact resistant than Kevlar. Other nano-architected materials have the capacity to refract light backward, a phenomenon not found in nature, and to create metamaterials that can be tuned to take on a variety of shapes. Known materials, such as a sponge, have the ability to take on different shapes depending on conditions: one shape when wet, another when dry. But these carefully architected lattices can be finely controlled to take on a variety of "in-between" states. Some nanoscale devices have benefited from machine learning, where multiple iterations of a design are printed out until the device is optimized to, for example, separate light by wavelength and polarization, a technology that could be added to cameras to increase their resolution and efficiency.
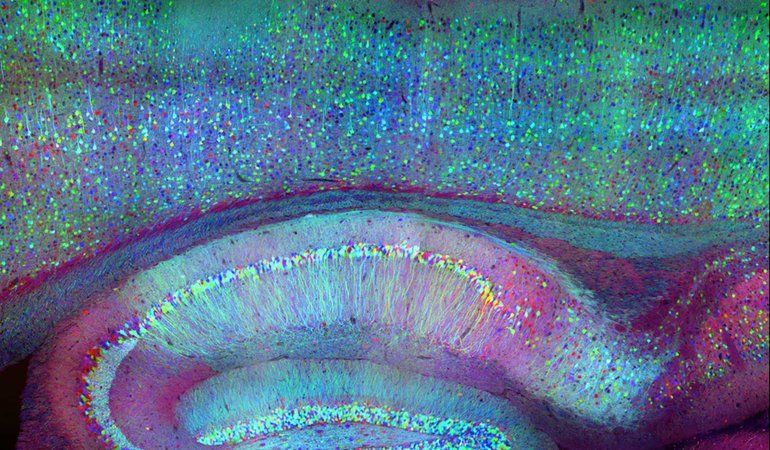
Understanding the Brain
Figuring out what is going on inside the brain is an enormous scientific challenge that draws researchers from many different disciplines. KNI founding director Michael Roukes, the Frank J. Roshek Professor of Physics, Applied Physics, and Bioengineering, has played a key role in organizing the effort, securing funding from President Barack Obama's BRAIN (Brain Research through Advancing Innovative Neurotechnology) Initiative in both 2014 and 2016, and organizing a TEDxCaltech event on the brain in 2013. Roukes's research has led to a method for mapping brain circuits called integrated photonics. Tiny optical microchips can be implanted inside the brain where they can be used to monitor neurons and also to control their activity. Prior to this, only brain circuits lying close to the surface of the brain could be monitored. Roukes's goal is to record the behavior of every neuron in a cubic millimeter of brain tissue (roughly 100,000 neurons).
Figuring out what is going on inside the brain is an enormous scientific challenge that draws researchers from many different disciplines. KNI founding director Michael Roukes, the Frank J. Roshek Professor of Physics, Applied Physics, and Bioengineering, has played a key role in organizing the effort, securing funding from President Barack Obama's BRAIN (Brain Research through Advancing Innovative Neurotechnology) Initiative in both 2014 and 2016, and organizing a TEDxCaltech event on the brain in 2013. Roukes's research has led to a method for mapping brain circuits called integrated photonics. Tiny optical microchips can be implanted inside the brain where they can be used to monitor neurons and also to control their activity. Prior to this, only brain circuits lying close to the surface of the brain could be monitored. Roukes's goal is to record the behavior of every neuron in a cubic millimeter of brain tissue (roughly 100,000 neurons).
Credit: Roukes et. al.