PASADENA, Calif.—Every great structure, from the Empire State Building to the Golden Gate Bridge, depends on specific mechanical properties to remain strong and reliable. Rigidity—a material's stiffness—is of particular importance for maintaining the robust functionality of everything from colossal edifices to the tiniest of nanoscale structures. In biological nanostructures, like DNA networks, it has been difficult to measure this stiffness, which is essential to their properties and functions. But scientists at the California Institute of Technology (Caltech) have recently developed techniques for visualizing the behavior of biological nanostructures in both space and time, allowing them to directly measure stiffness and map its variation throughout the network.
The new method is outlined in the February 4 early edition of the Proceedings of the National Academy of Sciences (PNAS).
"This type of visualization is taking us into domains of the biological sciences that we did not explore before," says Nobel Laureate Ahmed Zewail, the Linus Pauling Professor of Chemistry and professor of physics at Caltech, who coauthored the paper with Ulrich Lorenz, a postdoctoral scholar in Zewail's lab. "We are providing the methodology to find out—directly—the stiffness of a biological network that has nanoscale properties."
Knowing the mechanical properties of DNA structures is crucial to building sturdy biological networks, among other applications. According to Zewail, this type of visualization of biomechanics in space and time should be applicable to the study of other biological nanomaterials, including the abnormal protein assemblies that underlie diseases like Alzheimer's and Parkinson's.
Zewail and Lorenz were able to see, for the first time, the motion of DNA nanostructures in both space and time using the four-dimensional (4D) electron microscope developed at Caltech's Physical Biology Center for Ultrafast Science and Technology. The center is directed by Zewail, who created it in 2005 to advance understanding of the fundamental physics of chemical and biological behavior.
"In nature, the behavior of matter is determined by its structure—the arrangements of its atoms in the three dimensions of space—and by how the structure changes with time, the fourth dimension," explains Zewail. "If you watch a horse gallop in slow motion, you can follow the time of the gallops, and you can see in detail what, for example, each leg is doing over time. When we get to the nanometer scale, that is a different story—we need to improve the spatial resolution to a billion times that of the horse in order to visualize what is happening."
Zewail was awarded the 1999 Nobel Prize in Chemistry for his development of femtochemistry, which uses ultrashort laser flashes to observe fundamental chemical reactions occurring at the timescale of the femtosecond (one millionth of a billionth of a second). Although femtochemistry can capture atoms and molecules in motion, giving the time dimension, it cannot concurrently show the dimensions of space, and thus the structure of the material. This is because it utilizes laser light with wavelengths that far exceed the dimension of a nanostructure, making it impossible to resolve and image nanoscale details in tiny physical structures such as DNA .
To overcome this major hurdle, the 4D electron microscope employs a stream of individual electrons that scatter off objects to produce an image. The electrons are accelerated to wavelengths of picometers, or trillionths of a meter, providing the capability for visualizing the structure in space with a resolution a thousand times higher than that of a nanostructure, and with a time resolution of femtoseconds or longer.
The experiments reported in PNAS began with a structure created by stretching DNA over a hole embedded in a thin carbon film. Using the electrons in the microscope, several DNA filaments were cut away from the carbon film so that a three-dimensional, free-standing structure was achieved under the 4D microscope.
Next, the scientists employed laser heat to excite oscillations in the DNA structure, which were imaged using the electron pulses as a function of time—the fourth dimension. By observing the frequency and amplitude of these oscillations, a direct measure of stiffness was made.
"It was surprising that we could do this with a complex network," says Zewail. "And yet by cutting and probing, we could go into a selective area of the network and find out about its behavior and properties."
Using 4D electron microscopy, Zewail's group has begun to visualize protein assemblies called amyloids, which are believed to play a role in many neurodegenerative diseases, and they are continuing their investigation of the biomechanical properties of these networks. He says that this technique has the potential for broad applications not only to biological assemblies, but also in the materials science of nanostructures.
Funding for the research outlined in the PNAS paper, "Biomechanics of DNA structures visualized by 4D electron microscopy," was provided by the National Science Foundation and the Air Force Office of Scientific Research. The Physical Biology Center for Ultrafast Science and Technology at Caltech is supported by the Gordon and Betty Moore Foundation.
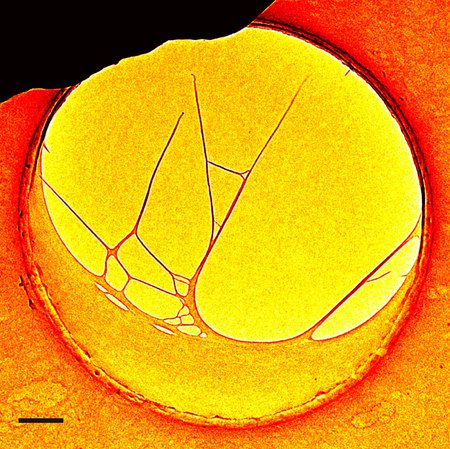
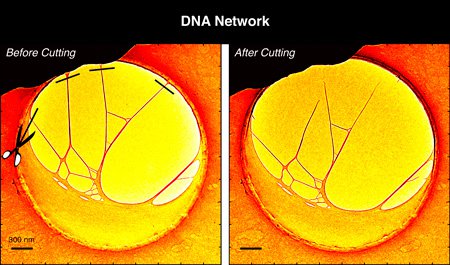
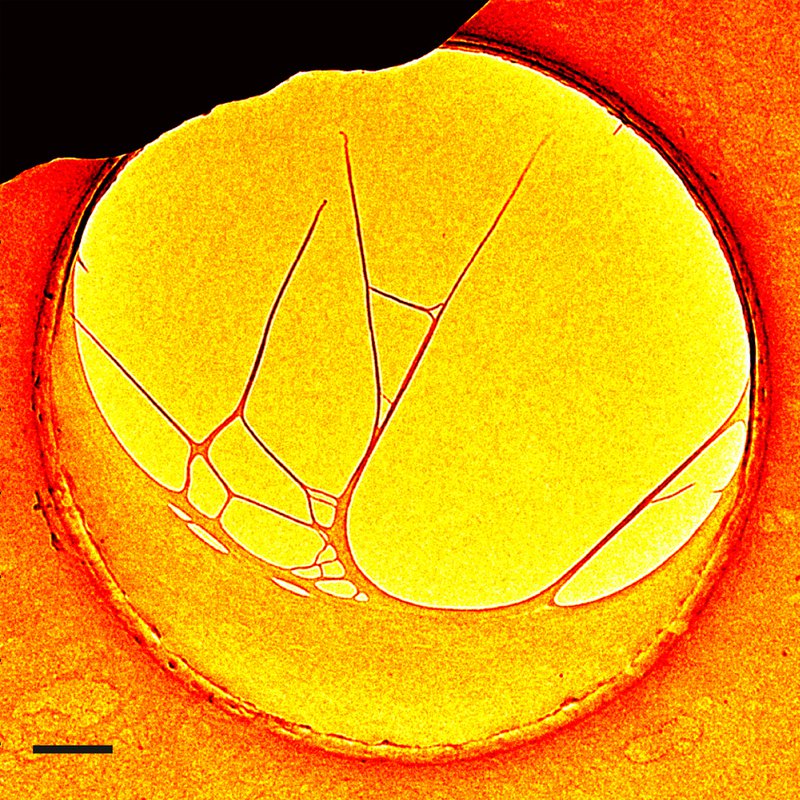